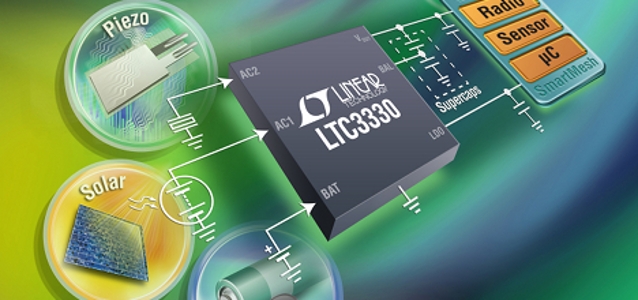
© Linear Technology
Application Notes |
What does all this energy harvesting stuff really mean?
The portable power application space is both broad and diverse. Products range from wireless sensor nodes (WSNs) that consume average power measured in microwatts to cart-based medical or data acquisition systems with multi-hundred Watt-hour battery packs.
Introduction
However, despite this variety, a few trends have emerged; namely, designers continue to demand more power in their products to support increased functionality and they want to charge the battery from any available power source. The first trend would imply increasing battery capacities. Unfortunately, users are often impatient and these increased capacities must be charged in a reasonable amount of time, which leads to increased charge currents. The second trend requires tremendous flexibility from the battery charging solution since they need to handle a broad range of input sources and power.
Fortunately, at the low end of the power spectrum are the nanopower conversion requirements of energy harvesting systems such as those commonly found in WSNs that necessitate the use of power conversion ICs, which deal in very low levels of power and current. These can be 10s of microwatts and nanoamps of current, respectively.
State-of-the-art and off-the-shelf energy harvesting technologies, for example in vibration energy harvesting and indoor photovoltaic cells, yield power levels on the order of milliwatts under typical operating conditions. While such power levels may appear restrictive, the operation of harvesting elements over a number of years can mean that the technologies are broadly comparable to long-life primary batteries, both in terms of energy provision and the cost per energy unit provided.
Moreover, systems incorporating energy harvesting are typically capable of recharging after depletion, something that systems powered by primary batteries cannot do. Nevertheless, most implementations will use an ambient energy source as the primary power source, but will supplement it with a primary battery that can be switched in if the ambient energy source goes away or is disrupted.
An Energy Harvesting WSN
There is plenty of ambient energy in the world around us and the conventional approach for energy harvesting has been through solar panels and wind generators. However, new harvesting tools allow us to produce electrical energy from a wide variety of ambient sources. Furthermore, it is not the energy conversion efficiency of the circuits that is important, but rather the amount of “average harvested” energy that is available to power it.
For instance, thermoelectric generators convert heat (or cold) into electricity, Piezo elements convert mechanical vibration, photovoltaics convert sunlight (or any photon source) and galvanics convert energy from moisture. This makes it possible to power remote sensors, or to charge a storage device such as a capacitor or thin film battery, so that a microprocessor or transmitter can be powered from a remote location without a local power source.
In general terms, the necessary IC performance characteristics needed for inclusion and use in the alternative energy market include the following:
Of course, the energy provided by the energy harvesting source depends on how long the source is in operation. Therefore, the primary metric for comparison of scavenged sources is power density, not energy density. Energy harvesting is generally subject to low, variable and unpredictable levels of available power so a hybrid structure that interfaces to the harvester and a secondary power reservoir is often used. The harvester, because of its unlimited energy supply and deficiency in power, is the energy source of the system.
The secondary power reservoir, either a battery or a capacitor, yields higher output power, but stores less energy, supplying power when required but otherwise regularly receiving charge from the harvester. Thus, in situations where there is no ambient energy from which to harvest power, the secondary power reservoir must be used to power the WSN. Of course, from system designers’ perspective, this adds a further degree of complexity since they must now take into consideration how much energy must be stored in the secondary reservoir to compensate for the lack of an ambient energy source. Just how much they will require will depend on several factors. These will include:
There are a plethora of applications where these power levels make sense for a system deployment. Here are just a few examples:
- Low standby quiescent currents – typically less than 6µA and as low as 450nA
- Low start-up voltages – as low as 20mV
- High input voltage capability – up to 34V continuous and 40V transients
- Ability to handle AC inputs
- Multiple output capability and autonomous system power management
- Auto-polarity operation
- Maximum Power Point Control (MPPC) for solar inputs
- The ability to harvest energy from as little as 1°C temperature delta
- Compact solution footprints with minimal external components
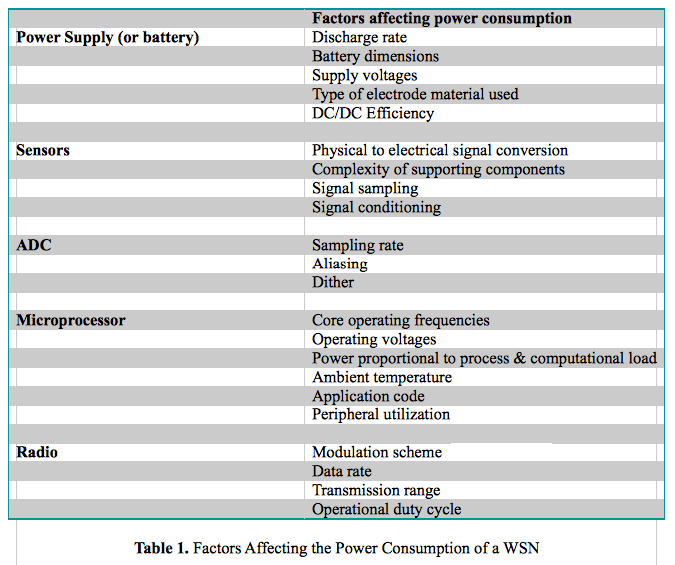
- The length of time the ambient energy source is absent
- The duty cycle of the WSN (that is the frequency with which a data reading and transmission has to be made)
- The size and type of secondary reservoir (capacitor, supercapacitor or battery)
- Is enough ambient energy available to act as both the primary energy source and have sufficient energy left over to charge up a secondary reservoir when it is not available for some specified period?
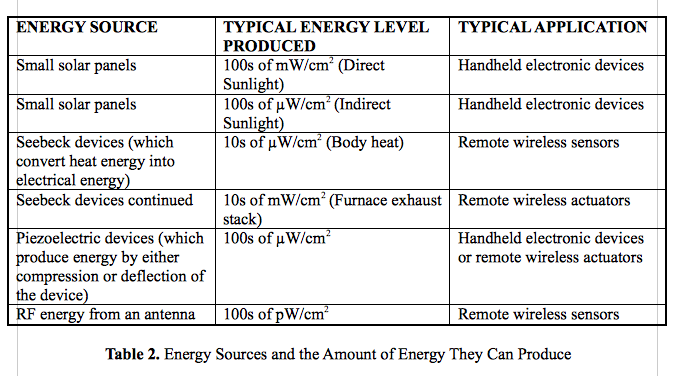
- Aircraft corrosion sensors
- Auto dimming windows
- Bridge monitors
- Building automation
- Electricity usage meters
- Gas sensors
- Health monitors
- HVAC controls
- Light switches
- Remote pipeline monitors
- Water meters
A Nanopower IC Solution It is clear that WSNs have very low levels of energy available. This, in turn, means that the components used in the system must be able to deal with these low power levels. While this has already been attained with the transceivers and microcontrollers, on the power conversion side of the equation, there has been a void. However, Linear Technology introduced its LTC3330 to specifically address this requirement The LTC3330 is a complete regulating energy harvesting solution that delivers up to 125mA of continuous output current to extend battery life when harvestable energy is available. It requires no supply current from the battery when providing regulated power to the load from harvested energy, and only 750nA, operating when powered from the battery under no-load conditions. The LTC3330 integrates a high voltage energy harvesting power supply, plus a synchronous buck-boost DC/DC converter when powered by a primary cell battery to create a single non-interruptible output for energy harvesting applications such as those in wireless sensor networks. The LTC3330’s energy harvesting power supply, consisting of a full-wave bridge rectifier accommodating AC or DC inputs and a high efficiency synchronous buck converter, harvests energy from piezoelectric (AC), solar (DC) or magnetic (AC) sources. The primary cell input powers a synchronous buck-boost converter that operates from 1.8V to 5.5V at its input, and can be used when harvested energy is not available to regulate the output whether the input is above, below or equal to the output. The LTC3330 automatically transitions to the battery when the harvesting source is no longer available. This has the added benefit of allowing the battery operated WSN to extend its operating life from 10 years to over 20 years if a suitable energy harvesting power source is available at least half of the time, and even longer if the energy harvesting source is more prevalent. This is significant since a Tadiran ‘C’ cell costs around $16 each. So the costs to swap them out with manpower are significant. Alternatively, a user could use a smaller (shorter life) battery and lower the overall system cost. Conclusion Even though portable applications and energy harvesting systems have a broad range of power levels for their correct operation, from microwatts to great than 1W, there are many power conversion ICs available for selection by the system designer. However, it is at the lower end of the power range, where nanoamps of currents need to be converted, where the choice becomes limited. Fortunately, the LTC3330 energy harvester and battery life extender, with its extremely low quiescent current makes it ideal for low power applications. A quiescent current of less than a microamp prolongs battery life for keep-alive circuits in portable electronics and enables a new generation of energy harvesting applications such as WSNs. ----- Author: Tony Armstrong, Director of Product Marketing at © Linear Technology Corporation-----
![]()